Oxygen's Complex Role in Retinopathy of Prematurity
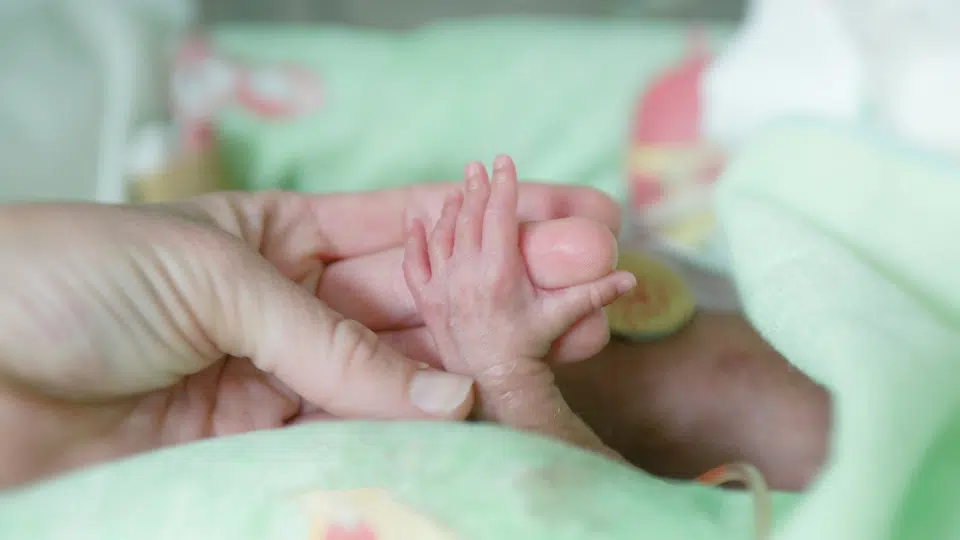
The Development of the Retina and ROP
Under usual circumstances, the retina’s blood vessels begin their formation around the fourth month of pregnancy and continue growing until the full-term due date. However, premature birth disrupts this natural timeline, leaving the fragile blood vessels in the retina susceptible to harm. Consequently, newly formed blood vessels can exhibit abnormalities. Often, these unusual vessels proliferate in unintended directions, frequently causing the detachment of the retina from the back of the eye (2). This process, known as retinal detachment, can lead to severe consequences, including vision loss and, in some cases, even blindness.
Risk for ROP is highest in babies born before 32 weeks of gestation or weighing less than 3 pounds at birth (2). While ROP might not initially present obvious symptoms, signs such as abnormal eye movements, white pupils (leukocoria), or vision issues can indicate its presence.
Amidst the vulnerability of these premature infants, a deeper understanding of the factors contributing to ROP becomes imperative. One of the most important elements is oxygen, which plays a vital role in supporting the growth of various tissues, including the retina (3). In the next section, we will discuss how the journey of premature birth often upsets the delicate balance of oxygen distribution and triggers a cascade of biological processes that drive ROP’s progression.
Oxygen’s Role in ROP and disease progression
The way oxygen influences the onset and progression of ROP can be summarized by the following sequence of events:
- Premature Birth: Premature infants are born before their retinas have fully developed. The retinal blood vessels start developing from the optic nerve and gradually extend toward the periphery of the retina. This sequence generally concludes during a full-term pregnancy. Yet, for preterm infants, this journey may remain unfinished, resulting in inadequate vascularization in the peripheral stretches of the retina.
- Oxygen Therapy: Premature infants frequently necessitate oxygen therapy owing to their underdeveloped pulmonary and respiratory systems. Yet, this necessary intervention can also disrupt the saturation of oxygen within the retina.
- Oxygen Toxicity: Surplus oxygen levels can bear detrimental effects on the maturation of blood vessels, including those within the retina. Elevated oxygen concentrations have the potential to induce vasoconstriction, a constriction of blood vessels that may subsequently diminish blood circulation to specific body regions, including the delicate retinal tissue (4).
- Retinal Vascular Development: The developing retinal blood vessels are responsive to fluctuations in oxygen concentrations. Abrupt shifts toward either hypoxia, insufficient oxygen, or hyperoxia, excessive oxygen, can markedly disrupt the typical course of vascularization. When oxygen levels are too high, blood vessel growth in the retina may be suppressed. Conversely, when oxygen levels drop, a compensatory growth of abnormal blood vessels can occur (5).
- Abnormal Vascularization: A distinctive feature of ROP is the aberrant proliferation of blood vessels in response to oxygen variations. This atypical development is marked by the emergence of delicate, permeable blood vessels that heighten the risk of retinal detachment, hemorrhaging, and scarring (5). Left unaddressed, these transformations can severely compromise vision and, in extreme cases, result in irreversible blindness.
As oxygenation and ROP are linked through a complex relationship, a pivotal aspect of effectively addressing ROP hinges on the ability to accurately measure oxygen saturation within the eye (6).
Care providers have expressed a pressing need for a noninvasive tool capable of capturing the subtleties of ROP’s early phases.
Encountering Limitations in ROP Diagnosis and Treatment
Recent years have witnessed the launch of several comprehensive clinical trials aimed at determining the optimal approach for administering oxygen to newborns to prevent ROP (7). While these endeavors provide valuable insights, they are not without their challenges.
At present, the pulse oximeter stands as the primary tool for gleaning information about ROP. Additionally, caregivers utilize more invasive methods such as periodic blood gas sampling from the baby’s heel to obtain further insight into its respiratory status. By employing these techniques, healthcare professionals can ascertain oxygen levels within the peripheral circulation. However, the capabilities of both techniques fall short when it comes to generating data related to ocular circulation.
Another avenue lies in novel imaging techniques. Utilizing methods such as intravenous fluorescein angiography (IVFA), optical coherence tomography (OCT), and OCT angiography (OCTA), medical practitioners have gained valuable insight into ROP’s origins, its progression, and its response to treatment (8). These tools undoubtedly offer valuable contributions, yet they are constrained in their ability to extend beyond identifying structural damage that impacts retinal tissues. Consequently, diagnoses often occur at later stages of disease advancement.
These challenges can prove significantly detrimental to the efforts aimed at preserving children’s vision. Care providers have expressed a pressing need for a noninvasive tool capable of capturing the subtleties of ROP’s early phases.
A Pathway to Personalized Treatment
A new and promising era of ROP management is introduced by a groundbreaking technology poised to offer early detection and monitoring: targeted ocular oximetry. This innovative approach is being developed to measure oxygenation levels within specified areas of the eye’s fundus, with the promise of providing unprecedented and valuable insights. The aim is to provide care providers an unobstructed window into the real-time oxygen activity where it truly matters – the back of the eye. With this leap forward, doctors may gain the capacity to precisely tailor ROP treatments in alignment with each infant’s unique requirements, potentially paving the way for better outcomes for the most vulnerable among us.
Conclusion
ROP casts a shadow over the sight of premature infants, risking vision loss or blindness. The role of oxygenation in the disease is complex and multifaceted, as both excess and deficiency of oxygen can trigger abnormal blood vessels growth in the retina. Current methods for measuring oxygen levels in the eye are either invasive, inaccurate, or limited in their scope. Therefore, there is a need for a noninvasive tool that can capture the subtleties of ROP’s early phases and provide real-time feedback on oxygen activity in the retina. Targeted ocular oximetry is a promising technology that may fill this gap and enable personalized ROP treatment. By providing new information about ROP’s mechanisms and progression, it has the potential to transform ROP care and improve outcomes for premature infants. This can make a world of difference for little eyes!
References
- Hong EH, Shin YU, Cho H. Retinopathy of prematurity: a review of epidemiology and current treatment strategies. Clin Exp Pediatr. 2022 Mar;65(3):115-126. doi: 10.3345/cep.2021.00773. Epub 2021 Oct 12. PMID: 34645255; PMCID: PMC8898617.
- Qayyum S. Development of retinopathy of prematurity. Community Eye Health. 2018;31(101):S3. PMID: 30275658; PMCID: PMC6157807.
- Sernagor, E., Eglen, S., Harris, B., & Wong, R. (Eds.). (2006). Retinal Development. Cambridge: Cambridge University Press. doi:10.1017/CBO9780511541629
- Hartnett ME, Lane RH. Effects of oxygen on the development and severity of retinopathy of prematurity. J AAPOS. 2013 Jun;17(3):229-34. doi: 10.1016/j.jaapos.2012.12.155. PMID: 23791404; PMCID: PMC3740273.
- Cavallaro, G., Filippi, L., Bagnoli, P., La Marca, G., Cristofori, G., Raffaeli, G., Padrini, L., Araimo, G., Fumagalli, M., Groppo, M., Dal Monte, M., Osnaghi, S., Fiorini, P. and Mosca, F. (2014), The pathophysiology of retinopathy of prematurity: an update of previous and recent knowledge. Acta Ophthalmologica, 92: 2-20. https://doi.org/10.1111/aos.12049
- Vehmeijer WB, Magnusdottir V, Eliasdottir TS, Hardarson SH, Schalij-Delfos NE, Stefánsson E. Retinal Oximetry with Scanning Laser Ophthalmoscope in Infants. PLoS One. 2016 Feb 3;11(2):e0148077. doi: 10.1371/journal.pone.0148077. PMID: 26840073; PMCID: PMC4740427.
- Stefánsson, E. (2011), How to prevent retinopathy of prematurity. Acta Ophthalmologica, 89: 3-4. https://doi.org/10.1111/j.1755-3768.2010.02089.x
- Chan RVP. Optical Coherence Tomography for Retinopathy of Prematurity and the Future of Retinopathy of Prematurity Screening. JAMA Ophthalmol. 2022;140(11):1127–1128. doi:10.1001/jamaophthalmol.2022.4326
Written by the Zilia Team on August 25, 2023
More on our Blog
Spectroscopy: From the Stars to the Eyes
The James Webb Space Telescope is the result of an international collaborative effort to solve mysteries in...
University of Houston Presents First Abstract on Ocular Oximetry
Diabetes is projected to affect 640 million adults worldwide by 2040. Diabetic retinopathy (DR), a retinal...
The Eye as a Window to AI in Healthcare
The term Artificial Intelligence (AI) was first coined by John McCarthy in 1956 when he defined the concept...
Solutions